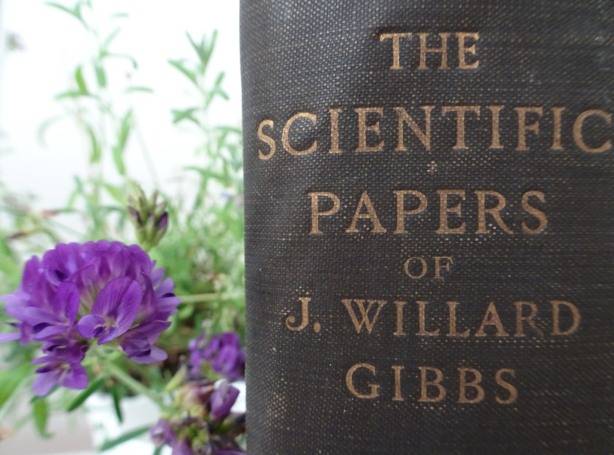
From the perspective of classical thermodynamics, osmosis has a rather unclassical history. Part of the reason for this, I suspect, is that osmosis was originally categorised under the heading of biology. I can remember witnessing the first practical demonstration of osmosis in a biology class, the phenomenon being explained in terms of pores (think invisible holes) in the membrane that were big enough to let water molecules through, but not big enough to let sucrose molecules through. It was just like a kitchen sieve, we were told. It lets the fine flour pass through but not clumps. This was very much the method of biology in my day, explaining things in terms of imagined mechanism and analogy.
And it wasn’t just in my day. In 1883, JH van ‘t Hoff, an able theoretician and one of the founders of the new discipline of physical chemistry, became suddenly convinced that solutions and gases obeyed the same fundamental law, pv = RT. Imagined mechanism swiftly followed. In van ‘t Hoff’s interpretation, osmotic pressure depended on the impact of solute molecules against the semipermeable membrane because solvent molecules, being present on both sides of the membrane through which they could freely pass, did not enter into consideration.
It all seemed very plausible, especially when van ‘t Hoff used the osmotic pressure measurements of the German botanist Wilhelm Pfeffer to compute the value of R in what became known as the van ‘t Hoff equation

where Π is the osmotic pressure, and found that the calculated value for R was almost identical with the familiar gas constant. There really did seem to be a parallelism between the properties of solutions and gases.

JH van ‘t Hoff (1852-1911)
The first sign that there was anything amiss with the so-called gaseous theory of solutions came in 1891 when van ‘t Hoff’s close colleague Wilhelm Ostwald produced unassailable proof that osmotic pressure is independent of the nature of the membrane. This meant that hypothetical arguments as to the cause of osmotic pressure, such as van ‘t Hoff had used as the basis of his theory, were inadmissible.
A year later, in 1892, van ‘t Hoff changed his stance by declaring that the mechanism of osmosis was unimportant. But this did not affect the validity of his osmotic pressure equation ΠV = RT. After all, it had been shown to be in close agreement with experimental data for very dilute solutions.
It would be decades – the 1930s in fact – before the van ‘t Hoff equation’s formal identity with the ideal gas equation was shown to be coincidental, and that the proper thermodynamic explanation of osmotic pressure lay elsewhere.
But long before the 1930s, even before Wilhelm Pfeffer began his osmotic pressure experiments upon which van ‘t Hoff subsequently based his ideas, someone had already published a thermodynamically exact rationale for osmosis that did not rely on any hypothesis as to cause.
That someone was the American physicist Josiah Willard Gibbs. The year was 1875.

J. Willard Gibbs (1839-1903)
– – – –
Osmosis without mechanism
It is a remarkable feature of Gibbs’ On the Equilibrium of Heterogeneous Substances that having introduced the concept of chemical potential, he first considers osmotic forces before moving on to the fundamental equations for which the work is chiefly known. The reason is Gibbs’ insistence on logical order of presentation. The discussion of chemical potential immediately involves equations of condition, among whose different causes are what Gibbs calls a diaphragm, i.e. a semipermeable membrane. Hence the early appearance of the following section
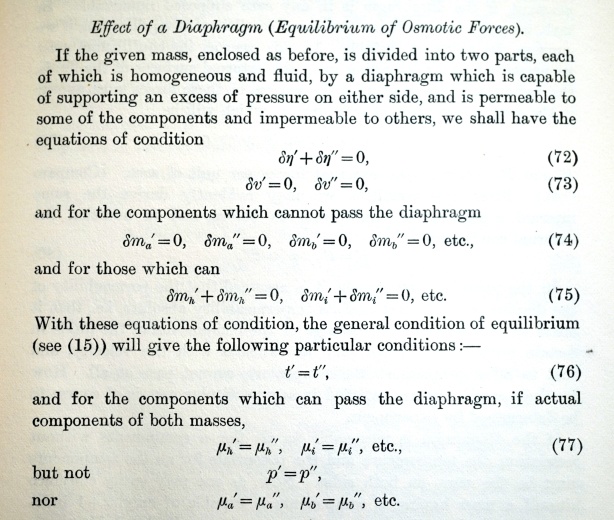
In equation 77, Gibbs presents a new way of understanding osmotic pressure. He makes no hypotheses about how a semipermeable membrane might work, but simply states the equations of condition which follow from the presence of such a membrane in the kind of system he describes.
This frees osmosis from considerations of mechanism, and explains it solely in terms of differences in chemical potential in components which can pass the diaphragm while other components cannot.
In order to achieve equilibrium between say a solution and its solvent, where only the solvent can pass the diaphragm, the chemical potential of the solvent in the fluid on both sides of the membrane must be the same. This necessitates applying additional pressure to the solution to increase the chemical potential of the solvent in the solution so it equals that of the pure solvent, temperature remaining constant. At equilibrium, the resulting difference in pressure across the membrane is the osmotic pressure.
Note that the increase in chemical potential of the solvent with increasing pressure is dependent on the partial molar volume of the solvent in the solution (V1) being positive since

There are however rare exceptions, where V1 is negative and the opposite happens. For more detail see my post “The Thermodynamics of Osmotic Pressure”.
– – – –
Europe fails to notice (almost)
Gibbs published On the Equilibrium of Heterogeneous Substances in Transactions of the Connecticut Academy. Choosing such an obscure journal (seen from a European perspective) clearly would not attract much attention across the pond, but Gibbs had a secret weapon. He had a mailing list of the world’s greatest scientists to which he sent reprints of his papers.
One of the names on that list was James Clerk Maxwell, who instantly appreciated Gibbs’ work and began to promote it in Europe. On Wednesday 24 May 1876, the year that ‘Equilibrium’ was first published, Maxwell gave an address at the South Kensington Conferences in London on the subject of Gibbs’ development of the doctrine of available energy on the basis of his new concept of the chemical potentials of the constituent substances. But the audience did not share Maxwell’s enthusiasm, or in all likelihood share his grasp of Gibbs’ ideas. When Maxwell tragically died three years later, Gibbs’ powerful ideas lost their only real champion in Europe.
It was not until 1891 that interest in Gibbs masterwork would resurface through the agency of Wilhelm Ostwald, who together with van ‘t Hoff and Arrhenius were the founders of the modern school of physical chemistry.

Wilhelm Ostwald (1853-1932) He not only translated Gibbs’ masterwork into German, but also produced a profound proof – worthy of Sadi Carnot himself – that osmotic pressure must be independent of the nature of the semipermeable membrane.
Although perhaps overshadowed by his colleagues, Ostwald had a talent for sensing the direction that the future would take and was also a shrewd judge of intellect – he instinctively felt that there were hidden treasures in Gibbs’ magnum opus. After spending an entire year translating ‘Equilibrium’ into German, Ostwald wrote to Gibbs:
“The translation of your main work is nearly complete and I cannot resist repeating here my amazement. If you had published this work over a longer period of time in separate essays in an accessible journal, you would now be regarded as by far the greatest thermodynamicist since Clausius – not only in the small circle of those conversant with your work, but universally—and as one who frequently goes far beyond him in the certainty and scope of your physical judgment. The German translation, hopefully, will more secure for it the general recognition it deserves.”
The following year – 1892 – another respected scientist sent a letter to Gibbs regarding ‘Equilibrium’. This time it was the British physicist, Lord Rayleigh, who asked Gibbs:
“Have you ever thought of bringing out a new edition of, or a treatise founded upon, your “Equilibrium of Het. Substances.” The original version though now attracting the attention it deserves, is too condensed and too difficult for most, I might say all, readers. The result is that as has happened to myself, the idea is not grasped until the subject has come up in one’s own mind more or less independently.”
Rayleigh was probably just being diplomatic when he remarked that Gibbs’ treatise was ‘now attracting the attention it deserves’. The plain fact is that nobody gave it any attention at all. Gibbs and his explanation of osmosis in terms of chemical potential was passed over, while European and especially British theoretical work centered on the more familiar and more easily understood concept of vapor pressure.
– – – –
Gibbs tries again
Although van ‘t Hoff’s osmotic pressure equation ΠV = RT soon gained the status of a law, the gaseous theory that lay behind it remained clouded in controversy. In particular, van ‘t Hoff’s deduction of the proportionality between osmotic pressure and concentration was an analogy rather than a proof, since it made use of hypothetical considerations as to the cause of osmotic pressure. Following Ostwald’s proof that these were inadmissible, the gaseous theory began to look hollow. A better theory was needed.

Lord Kelvin (1824-1907) and Lord Rayleigh (1842-1919)
This was provided in 1896 by the British physicist, Lord Rayleigh, whose proof was free of hypothesis but did make use of Avogadro’s law, thereby continuing to assert a parallelism between the properties of solutions and gases. Heavyweight opposition to this soon materialized from the redoubtable Lord Kelvin. In a letter to Nature (21 January 1897) he charged that the application of Avogadro’s law to solutions had “manifestly no theoretical foundation at present” and further contended that
“No molecular theory can, for sugar or common salt or alcohol, dissolved in water, tell us what is the true osmotic pressure against a membrane permeable to water only, without taking into account laws quite unknown to us at present regarding the three sets of mutual attractions or repulsions: (1) between the molecules of the dissolved substance; (2) between the molecules of water; (3) between the molecules of the dissolved substance and the molecules of water.”
Lord Kelvin’s letter in Nature elicited a prompt response from none other than Josiah Willard Gibbs in America. Twenty-one years had now passed since James Clerk Maxwell first tried to interest Europe in the concept of chemical potentials. In Kelvin’s letter, with its feisty attack on the gaseous theory, Gibbs saw the opportunity to try again.
In his letter to Nature (18 March 1897), Gibbs opined that “Lord Kelvin’s very interesting problem concerning molecules which differ only in their power of passing a diaphragm, seems only to require for its solution the relation between density and pressure”, and highlighted the advantage of using his potentials to express van ‘t Hoff’s law:
“It will be convenient to use certain quantities which may be called the potentials of the solvent and of the solutum, the term being thus defined: – In any sensibly homogeneous mass, the potential of any independently variable component substance is the differential coefficient of the thermodynamic energy of the mass taken with respect to that component, the entropy and volume of the mass and the quantities of its other components remaining constant. The advantage of using such potentials in the theory of semi-permeable diaphragms consists partly in the convenient form of the condition of equilibrium, the potential for any substance to which a diaphragm is freely permeable having the same value on both sides of the diaphragm, and partly in our ability to express van’t Hoff law as a relation between the quantities characterizing the state of the solution, without reference to any experimental arrangement.”
But once again, Gibbs and his chemical potentials failed to garner interest in Europe. His timing was also unfortunate, since British experimental research into osmosis was soon to be stimulated by the aristocrat-turned-scientist Lord Berkeley, and this in turn would stimulate a new band of British theoreticians, including AW Porter and HL Callendar, who would base their theoretical efforts firmly on vapor pressure.
– – – –
Things Come Full Circle
As the new century dawned, van ‘t Hoff cemented his reputation with the award of the very first Nobel Prize for Chemistry “in recognition of the extraordinary services he has rendered by the discovery of the laws of chemical dynamics and osmotic pressure in solutions”.
The osmotic pressure law was held in high esteem, and despite Lord Kelvin’s protestations, Britain was well disposed towards the Gaseous Theory of Solutions. The idea circulating at the time was that the refinements of the ideal gas law that had been shown to apply to real gases, could equally well be applied to more concentrated solutions. As Lord Berkeley put it in the introduction to a paper communicated to the Royal Society in London in May 1904:
“The following work was undertaken with a view to obtaining data for the tentative application of van der Waals’ equation to concentrated solutions. It is evidently probable that if the ordinary gas equation be applicable to dilute solutions, then that of van der Waals, or one of analogous form, should apply to concentrated solutions – that is, to solutions having large osmotic pressures.”
Lord Berkeley’s landmark experimental studies on the osmotic pressure of concentrated solutions called renewed attention to the subject among theorists, who now had some fresh and very accurate data to work with. Alfred Porter at University College London attempted to make a more complete theory by considering the compressibility of a solution to which osmotic pressure was applied, while Hugh Callendar at Imperial College London combined the vapor pressure interpretation of osmosis with the hypothesis that osmosis could be described as vapor passing through a large number of fine capillaries in the semipermeable membrane. This was in 1908.

H L Callendar (1863-1930)
So seventeen years after Wilhelm Ostwald conclusively proved that hypothetical arguments as to the cause of osmotic pressure were inadmissible, things came full circle with hypothetical arguments once more being advanced as to the cause of osmotic pressure.
And as for Gibbs, his ideas were as far away as ever from British and European Science. The osmosis papers of both Porter (1907) and Callendar (1908) are substantial in referenced content, but nowhere do either of them make any mention of Gibbs or his explanation of osmosis on the basis of chemical potentials.
There is a special irony in this, since in Callendar’s case at least, the scientific papers of J Willard Gibbs were presumably close at hand. Perhaps even on his office bookshelf. Because that copy of Gibbs’ works shown in the header photo of this post – it’s a 1906 first edition – was Hugh Callendar’s personal copy, which he signed on the front endpaper.

Hugh Callendar’s signature on the endpaper of his personal copy of Gibbs’ Scientific Papers, Volume 1, Thermodynamics.
– – – –
Epilogue
Throughout this post, I have made repeated references to that inspired piece of thinking by Wilhelm Ostwald which conclusively demonstrated that osmotic pressure must be independent of the nature of the membrane.
Ostwald’s reasoning is so lucid and compelling, that one wonders why it didn’t put an end to speculation on osmotic mechanisms. But it didn’t, and hasn’t, and probably won’t.
Here is how Ostwald presented the argument in his own Lehrbuch der allgemeinen Chemie (1891). Enjoy.

“… it may be stated with certainty that the amount of pressure is independent of the nature of the membrane, provided that the membrane is not permeable by the dissolved substance. To understand this, let it be supposed that two separating partitions, A and B, formed of different membranes, are placed in a cylinder (fig. 17). Let the space between the membranes contain a solution and let there be pure water in the space at the ends of the cylinder. Let the membrane A show a higher pressure, P, and the membrane B show a smaller pressure, p. At the outset, water will pass through both membranes into the inner space until the pressure p is attained, when the passage of water through B will cease, but the passage through A will continue. As soon as the pressure in the inner space has been thus increased above p, water will be pressed out through B. The pressure can never reach the value P; water must enter continuously through A, while a finite difference of pressures is maintained. If this were realised we should have a machine capable of performing infinite work, which is impossible. A similar demonstration holds good if p>P ; it is, therefore, necessary that P=p; in other words, it follows necessarily that osmotic pressure is independent of the nature of the membrane.”
(English translation by Matthew Pattison Muir)
– – – –
P Mander July 2015